There seems to be agreement from all sides that hydrogen is the fuel of the future. It is combustible and energy rich, and because its molecular formula is all H, there is no carbon in the equation to emit. If our utility-scale electricity on the grid, industrial and commercial heat, and even transportation sectors can utilized hydrogen in place of traditional hydrocarbons, it seems we could decarbonize immediately.
But what does the on-ramp really look like? The answer summons many players, including engineers and physicists, construction workers and urban planners, and even public policy officials at all levels.
The first challenge is producing hydrogen, because it is not naturally occurring. For this, there are a number of options, each packing its own set of ticking clocks until the molecule has a decarbonizing impact. Among the methods are coal gasification and steam methane reforming – each of which utilize hydrocarbons and emit carbon dioxide in the process. These are disfavored because they add carbon to the atmospheric balance to make the hydrogen and therefore step forward and backward at the same time. Even when paired with carbon capture to reduce emissions by half or more becoming “blue hydrogen,” these options create a very long, slow ramp to decarbonization.
Another reason for the slow ramp is the centralized nature of production. By producing hydrogen at an industrial level for use around the country, new infrastructure must be developed. In other words, even if we had pure hydrogen that popped into existence in the middle of the country, we could not switch it out with methane or other fuels to decarbonize in an instant. We will need to build new specialized hydrogen pipelines, storage tanks, vehicle tanks, and other production, transportation, and storage components. These too are material-intensive and energy-intensive, and with permitting for any infrastructure project taking at least three to five years, this puts the decarbonization benefit a decade into the future.
This same problem also impacts the preferred production method of hydrogen: green hydrogen. To produce green hydrogen, electrolysis is used to separate the hydrogen from water molecules using energy from zero or low carbon energy sources like wind, solar, geothermal, hydropower, or nuclear. Unlike grey or blue hydrogen produced by extracting hydrogen off of coal or methane, green hydrogen from water and renewable power puts the least carbon into the atmosphere and preserves its decarbonizing steps forward. But as above, this too, if produced centralized and at scale, will come with buildouts that will add time and carbon into the atmosphere, slowing the ultimate effect of decarbonization.
A less discussed option that short circuits some of these concerns is decentralized or distributed production, and in particular methane pyrolysis. While this does use methane as its initial molecule from which to extract hydrogen, it pulls the carbon out on the front end. This pre-combustion carbon capture results in power carbon, effectively decarbonizing natural gas and sending only hydrogen into combustion chambers for electricity or heat. This point of use hydrogen production means little or no retrofitting or new infrastructure. It also uses three times less electricity than electrolysis, meaning less strain on the grid and less demand for energy coming from fossil fuels or renewables.
But the picture is still not quite clear enough to simply flip a switch and generate all clean power from hydrogen. As researchers and critics point out, hydrogen use at natural gas utilities and similar industrial and commercial applications utilize blending. Rather than switch the input from methane to hydrogen overnight, the technique is to dial up the proportion of hydrogen over time. Yet one more delay is notable here before we achieve full decarbonization – the rate of change in carbon emissions at different fuel ratios.
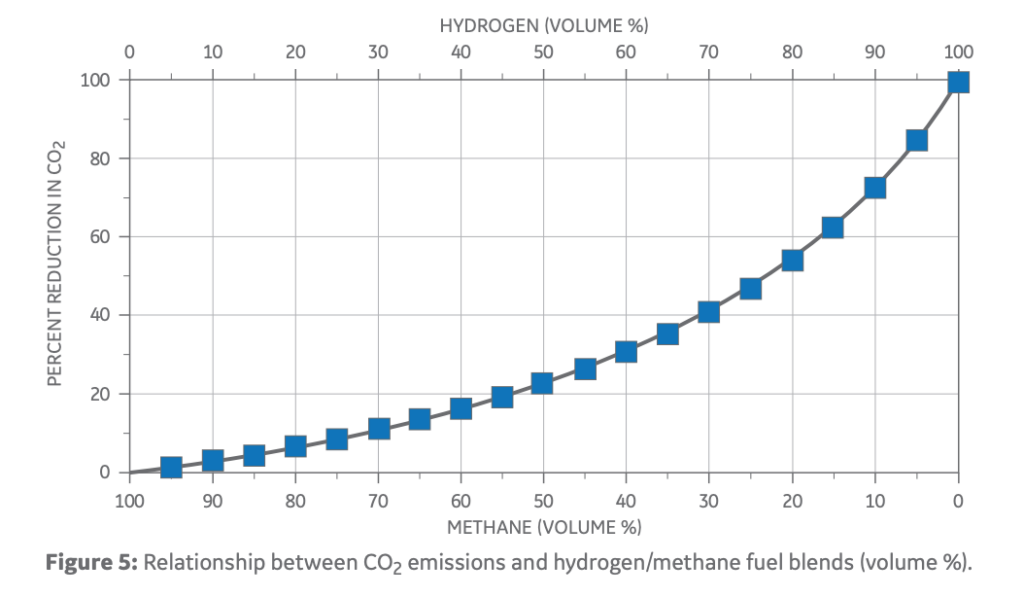
Rather than a linear relationship, where a 10 percent blend of hydrogen results in a 10 percent reduction in carbon dioxide, the volumetric blend curve takes time to ramp up. The pace at which utilities and heat users increase these blends may depend in part on public policy and political will. If these are initiated by industry and pledged by utilities, they should be clear commitments with well-articulated timelines and public accountability. Otherwise, the blends may not shift overtime.
At the end of the day, public policy is a critical aspect of decarbonization through hydrogen. While it is the job of chemists, physicists, engineers, and other scientists to develop the technology to facilitate hydrogen production most efficiently – whether through centralized green hydrogen or distributed pyrolysis production – it is incumbent on public officials, lawmakers, and regulators to set the rules and streamline the processes.
Public policy will impact the tax, subsidy, and grants that enable hydrogen production. It will impact the permitting and approvals for new facilities, material handling, transportation options, infrastructure buildouts, and storage options. Public policy may even be used to specify targets for fuel uses, blends, and more – which may be set at the local, state, or federal levels.
To return to the initial question – how soon can hydrogen help decarbonize the entire economy – the answer is complicated. It will depend on the type of hydrogen production, whether it is centralized or distributed, the infrastructure needed to facilitate it, and the public policy rules that govern it. Those looking to hydrogen should not be discouraged by all these factors, but see with clear eyes where reforms will be needed to achieve the desired decarbonized future.
Written by Benjamin Dierker, Executive Director
The Alliance for Innovation and Infrastructure (Aii) is an independent, national research and educational organization. An innovative think tank, Aii explores the intersection of economics, law, and public policy in the areas of climate, damage prevention, energy, infrastructure, innovation, technology, and transportation.